This novel chip-scale electrochemical double-layer (ECDL) supercapacitor uses carbon nanotubes to provide high-power energy storage for low-volume and low-weight microelectronics systems. Traditional ECDL approaches that utilize activated carbon often have limitations imposed by the structure of their pores. Georgia Tech’s nanostructured carbon–based ECDL electrodes offer improved performance due to their high surface area, high conductivity, increased porosity, and options for functionalization that enhance pseudocapacitance (i.e., electrochemical storage of electricity).
Utilizing 3D space, Georgia Tech’s capacitor is built between two metal contacts. Arrays of graphene functionalized carbon nanotubes are grown within two etched silicon wafers that are fixed to each other. The wafers form an enclosed capacitive cell filled with electrolyte and carbon nanotubes that are firmly adhered to a metallic conduction layer and interconnected with the wafer-scale circuitry. This graphene-based technology can hold the equivalent energy of a lithium battery, addressing the rising need for high-energy capacitors.
These nanostructured ECDL capacitors can potentially meet the large-scale energy needs of electric vehicles, electromechanical actuators, and portable power supplies as well smaller scale needs of microelectronics systems.
- Decreased size: Minimizes space that does not contribute to volumetric density and is drastically smaller than conventional ECDL capacitors, allowing capacitor-dependent devices to shrink considerably
- High power: Achieves gravimetric energy densities of over 100 watt-hours per kilogram
- Improved performance: The vertically aligned graphene-functionalized carbon nanotubes Offers high porosity and surface area for improved pseudocapacitance by vertically aligning graphene-functionalized nanotubes
- Increased capacity: Uses atomic layer deposition processes that can create conformal pseudocapacitive coatings to greatly increase overall capacity
- Simple fabrication: Uses process that is compatible with traditional microelectronics approaches
- Versatile: Enables structures to be constructed in virtually any manner suitable for application to microelectronics
Small-scale energy needs (e.g., microelectronics systems):
- Pulsed wireless communication
- Embedded sensors
- Smart structures
- Wearable electronics and smart textiles
Large-scale energy needs:
- Electric vehicles
- Electromechanical actuators
- Portable power supplies
Over the last decade, electronics have made a shift from micro- to nano-scale devices. With the increase in miniaturization, electrical components such as resistors, batteries, and capacitors have been modified and redeveloped to fit the physical and mechanical characteristics of nano-scale electrical environments. The increased need for devices with high-energy storage and fast energy discharge capabilities has increased the demand for miniaturized high-energy capacitors.
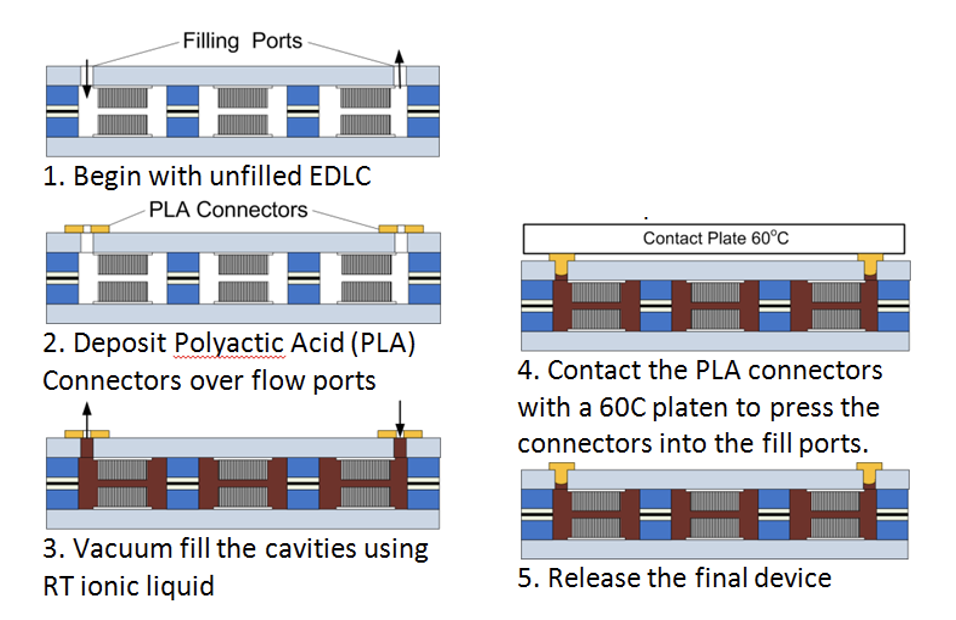
Baseline process flow for placing and sealing electrolyte within the micro-capacitor
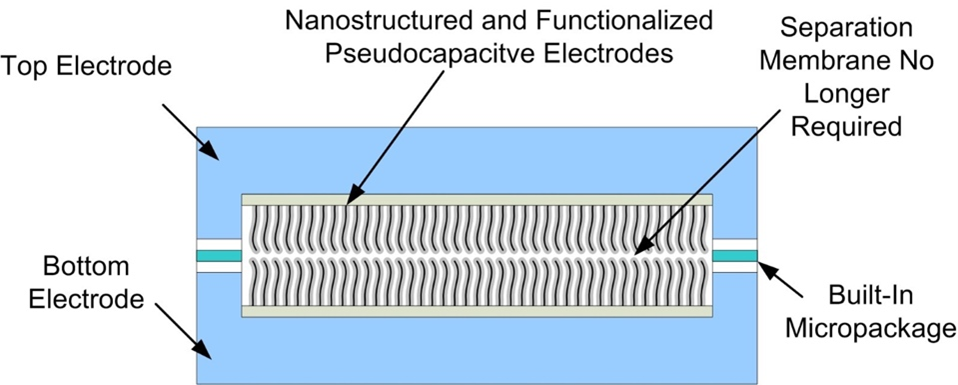
A vertically stacked ECDL structure.