Current electronic devices used in applications such as wireless acoustic communication, biosensing, and microfluidic sensing have limited power and are limited in the environments in which they can operate. For example, typical implantable medical devices are equipped with non-rechargeable batteries that last a lifetime, but that longevity results in limited power that fails to meet energy needs for some types of implants, such as those for deep brain stimulation, optogenetics, and peripheral nerve stimulation. Many methods of energizing these microdevices have been explored but are limited by low power densities, limited power transfer range, size, or tissue safety. Capacitive transducers typically require a DC bias or electret in order to operate efficiently and with high sensitivity, which makes them less desirable for wireless power transfer.
Another challenge is the application of mechanical frequency combs to multiple environments. While optical frequency combs have many applications, they cannot be used in water and biological fluids. Current mechanical/acoustic frequency comb generation uses purely mechanical resonators, which limits it to use in low loss conditions such as in air and vacuums.
New class of acoustic transducers enables efficient wireless power transfer and generation of frequency combs in different environments
This new class of transducers utilizes parametric resonance as a means for transduction. It transfers energy from the mechanical domain in the form of acoustic/ultrasonic/phononic waves to the electrical domain by modulating either the reactance or resistance component of an electrical resonator, such as an RLC (resistor/inductor/capacitor) circuit.
A capacitive micromachined ultrasonic transducer can be used as a pump for a parametric resonator to convert acoustic energy from an ultrasonic source to a sustained electrical signal, which can power implantable biomedical devices, small wearable sensors, and other wireless charging applications. Parametric resonance-based capacitive transducers also offer an alternative to traditional capacitive transducers as they can be operated without the need for a bias voltage or a pre-charged electret.
Using the same approach, simple yet robust generation of stable electromechanical frequency combs is also possible in both air and liquid environments using a capacitive micro-resonator array. By combining the mechanical resonator with an electrical oscillator, the frequency comb generation mechanism is isolated from the environmental conditions, enabling generation in liquid media. The system can be used as a highly sensitive sensor to detect small changes in force or fluid properties in the surrounding environment. This enables its practical use in applications such as gas sensing, underwater distance measurement, communication, and microfluidic sensing.
- Broadens applications: Frequency comb technology can be expanded to applications such as microfluidics and underwater acoustics.
- Extends environments: This technology enables use of the transducers and the generation of phononic frequency combs even in wet environments.
- Eliminates DC bias: The capacitive parametric ultrasonic transducer (CPUT) overcomes the DC bias requirement by driving the capacitive transducer into parametric resonance using ultrasound.
- Higher power: Use of ultrasound for biomedical implants means a higher power limit and better penetration into the human body.
- High sensitivity: This frequency comb system can detect small changes in force or fluid properties in the surrounding environment.
- Simplifies production: The capacitive ultrasonic transducer and resonance circuit can be fabricated by standard micromachining techniques with integration onto a semiconductor substrate.
Possible uses in both air and liquid environments for:
Power:
- Wireless power transfer and sensing for implantable devices such as biomedical implants and pacemakers
- Wireless charging of cell phones and other small wearable sensors for Internet of Things (IoT) applications
Sensing:
- Biosensing
- Microfluidic sensing
- Gas sensing
- Underwater distance measurement
Communication
- Wireless acoustic communication
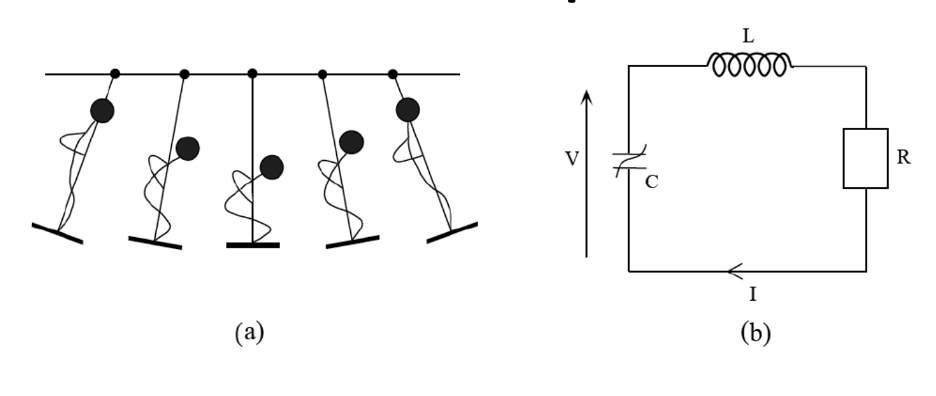
(a) A swing driven by a person to illustrate the concept of parametric oscillation. (b) The same concept shown in a circuit. Changing the capacitance in an RLC resonator (fo) circuit at 2fo frequency pumps energy to the circuit at fo. Energy is collected over R.