Quantitative optimization method needed to overcome tedious experimental tasks and application limitations
Oblique back-illumination microscopy (OBM) and quantitative OBM (qOBM) have greatly improved optical phase imaging systems by facilitating label-free phase contrast in thick scattering samples with epi-illumination. However, OBM/qOBM still requires onerous experimental processes by highly skilled technicians to optimize configurations. Furthermore, the resulting configurations are limited to only a few selections that are not universal (e.g., conditions, devices).
Optimized SNR enables OBM/qOBM to produce reliable results across multiple tissue types at a faster rate
Systematically identifying the detectable phase contrast signal-to-noise (SNR) ratio for a large parameter enables multiple non-intuitive geometries to be determined that will optimize any OBM/qOBM device in arbitrary conditions. This innovative framework lifts the constraints for OBM/qOBM configuration by human-in-the-loop adjustments. It uses acute phase SNR optimization computations to produce multiple optimized geometries for configuration much faster. Quantitively enhanced SNR enables in-silico optimization of a wide parameter space that is useful for in-vivo and in-situ measurements. Progress in finding an OBM/qOBM’s best geometries has resulted in SNR variations displaying reliable behavior (e.g., a sensitivity of <20 nanometers for a single qOBM capture) across different types of tissues with different scattering properties. These ideal parameters lead to optimal imaging conditions that allow for a universal application of a single device, whether fiber-based or not, to be used to study a variety of tissue types. With the opportunity for faster precision, optical phase imaging systems are one step closer to real-time intra-surgical use for surgery guidance, endoscopy, microscopy and histological analysis.
- Simpler and Faster: SNR optimization removes the arduous experimental configuration identification while computationally discovering both intuitive and non-intuitive geometries at a faster output rate.
- Reliable: A quantitatively enhanced SNR has shown reliability of in-vivo and in-situ measurements across multiple types of tissue (e.g., grey matter, white matter, epidermis, breast tissue).
- Universal: This method discovers more configurations that can work with any OBM/qOBM devices, whether they are fiber-based or not, in arbitrary environments as well as other optical phase imaging techniques.
This technology is applicable to multiple imaging systems used for:
- Surgical guidance
- Endoscopy
- Microscopy
- Histological analysis
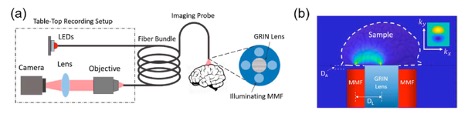
(a) Optical setup for measuring the quantitative phase using a fiber-based imaging probe. The probe design, which includes illuminating multimode fibers (MMF) and gradient index (GRIN) lenses, is optimized to achieve the high SNR ratio of retrievable brain contrast. (b) Epi-illumination geometry is optimized for the full quantitative phase information to be obtained. Figure adapted from "A Universal Approach to Optimize the Design of an Imaging Probe for Quantitative Oblique Back-illumination Microscopy." Microscopy Histopathology and Analytics. Optica Publishing Group, 2022.
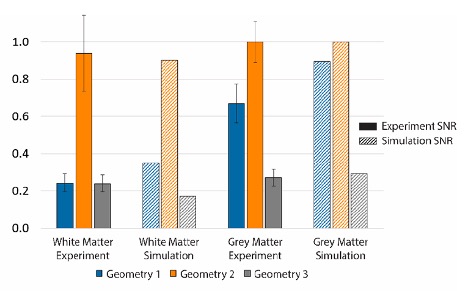
A comparison of experiment and simulation SNR values of three geometries with white matter and grey matter scattering phantoms that shows the SNR values are in good agreement across configurations and tissue types. Figure adapted from "Optimization of a flexible fiber-optic probe for epi-mode quantitative phase imaging." Optics Express 30.11 (2022): 17713-17729.