Two new methods developed by researchers at Georgia Tech to address measurement errors in quantum computers are resulting in a threefold improvement in measurement reliability. Quantum computers are known to be susceptible to errors, particularly measurement operations, and do not currently have the capacity to perform error correction.
Measurement errors have significant bias depending on the state being measured, with some states significantly more error-prone than other states. Therefore, the researchers first developed an “Invert and Measure” method that exploits the bias in measurement errors by performing an inversion before measurement if the state being measured is likely an error-prone state. Because it is not usually known if the system is in a weak state or strong state, Georgia Tech researchers developed two approaches.
First, a Static Invert-and-Measure (SIM) approach executes two instances of the program—one with standard measurements and the other with inverted measurements—and then combines the results. A second approach, Adaptive Invert and Measure (AIM), tailors the inversion profile to suit the machine characteristics and maps the likely solutions to be read in the strong state that is specific to the machine.
The second method mitigates correlated errors seen in quantum computers to reduce incorrect output during measurement. Rather than using a single mapping for all trials, this method, called the Ensemble of Diverse Mapping (EDM), splits the trials into groups and applies a diverse mapping to each group. A Weighted Ensemble of Diverse Mappings approach improves reliability further by placing different weights to the output produced by each mapping.
- Increased reliability by 2-3x: Some current quantum computers have average measurement error rates ranging from 6%–8%, with worst case rates ranging from 25%–30%. Georgia Tech’s methods improve measurement fidelity by 2–3x.
- Improved usability: Implementation of the EDM uses the top-four mappings produced by the underlying mapping policy.
- Proactive: Using AIM to predict the state that is being measured and the error rate profile of the machine, the predicted state can be proactively mapped to the strongest state using a specifically designed inversion string.
The applications for quantum computing are vast and include:
- Optimization
- Research
- Cryptography/Espionage
Industries that may be impacted most significantly include:
- Finance
- Materials science
- Healthcare (e.g., cancer treatments)
- Artificial intelligence
Quantum computers have the potential to solve hard problems that are beyond the capabilities of conventional computers. While quantum computers with several dozen qubits have already been demonstrated, machines with millions of qubits are unlikely to be available within the next decade. One of the biggest challenges in building a large-scale quantum computer is its vulnerability to errors.
The qubit devices quantum computers currently use are extremely susceptible to noise and cannot hold the state for more than several tens of microseconds. One of the ways they can tolerate noise is by using quantum error correction codes. Unfortunately, quantum error correction requires about 20–50 qubits to build one fault-tolerant logical qubit, making it likely to become viable only once systems have thousands of qubits. In the near future, Noisy Intermediate Scale Quantum (NISQ) machines with hundreds of noisy qubits will likely be operating in a noisy environment without any error correction.
Measurement is typically the most error-prone operation on a quantum computer, with current machines reporting errors ranging from 8% to 30%. While addressing how to mitigate measurement errors, the Georgia Tech researchers observed a state-dependent bias, with some states experiencing significantly higher error rates compared to the other states.
The applications on a NISQ machine try to estimate the correct output by running the same program thousands of times and logging the results. If the error rates are low and the errors are not correlated, then the correct answer can be inferred as the one appearing with the highest frequency. Unfortunately, quantum computers are vulnerable to correlated errors, which can cause an incorrect answer to appear more frequently than the correct answer.
This technology addresses both state-dependent bias and correlated errors.
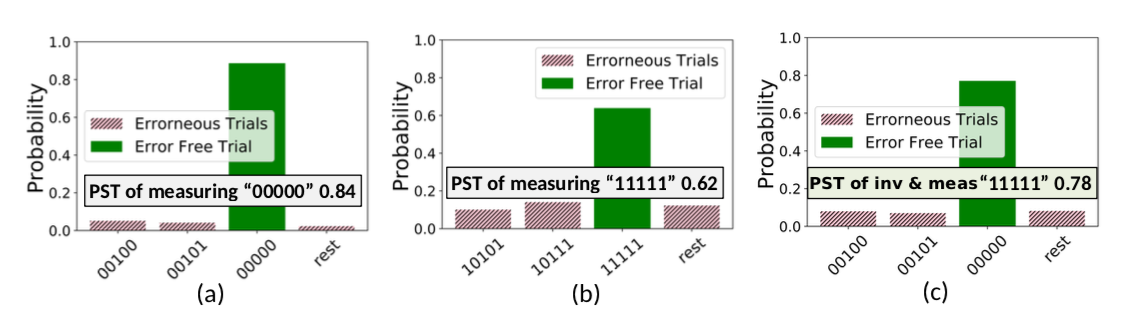
Probability of successfully measuring a state (a) All-zero state "00000" (b) All-ones state "11111" (c) Measuring the All-ones state by first inverting the state and then performing the measurement (expected output "00000").
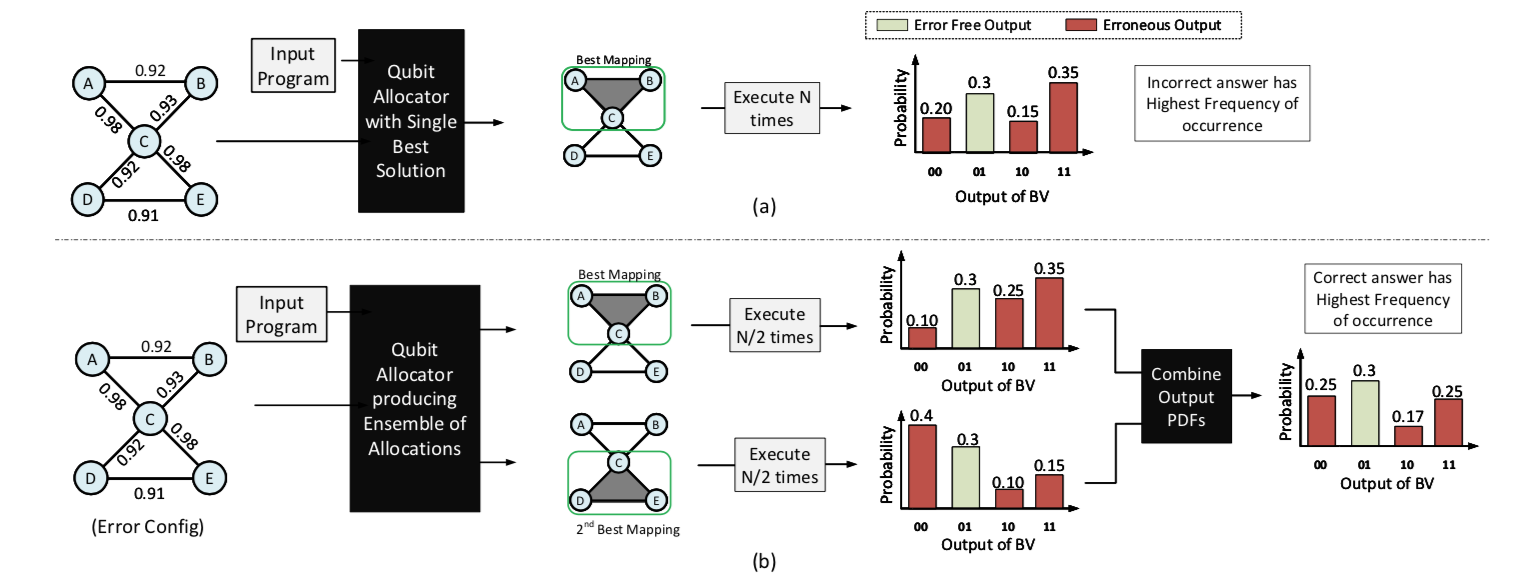
Bernstein-Vazirani using (a) Single best mapping and (b) Ensemble of Diverse Mappings (EDM), running two allocations and merging the outputs (EDM infers correct answer even if both mappings produce incorrect answer).